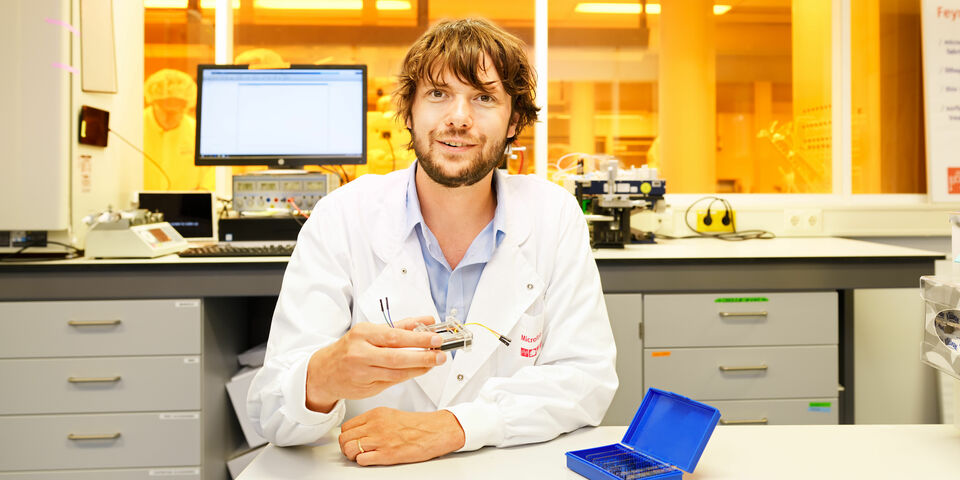
- Research
- 16/06/2020
Artificial synapse 'talks' to living cells
Scientists at TU/e led by Yoeri van de Burgt, together with American and Italian researchers, have succeeded in developing artificial synapses that can communicate with living cells. This system may be used in the future to 'connect' prostheses to the brain. The results have been published in Nature Materials.
The brain consists of nerve cells that send electrochemical signals (neurotransmitters and ions) to each other. These cells talk to each other through two synapses and a narrow cleft in between that serves, as it were, the means to transport the signals. Each time a signal passes through the synaptic cleft, the connection becomes stronger and the transmission expends less energy. This is because the signals permanently adjust the conductivity of the receiving synapse. This strengthening of the path is the way the brain learns. The receiving synapse not only processes the signals, it also has a memory function. All this makes it an ultra-efficient and learning system.
In 2017 TU/e researcher Yoeri van de Burgt, then postdoc at Stanford University, successfully developed an artificial synapse made of organic materials. With the same research team he has now been able to make this synapse actually communicate with living cells that resemble nerve cells. Van de Burgt: "Just like a real brain, our system appears to have a learning and a memory function. This brings us one step closer to an adaptive connection with the brain, which makes advanced prostheses and regenerative medicine possible.”
Learning process as in a real brain
"Most research groups that work on measuring brain activity and brain-machine interfaces are only able to measure electrical signals. But those signals are only a derivative of the processes in the synapse. We can really mimic the process. We work, just like the brain itself, with electrochemical signals. That makes our approach more efficient but also more relevant," says Van de Burgt.
The system of two synapses and a synaptic cleft was reconstructed by the researchers using two conductive electrodes consisting of a soft polymer and an electrolyte solution in between. Subsequently, the researchers were able to stick the living cells on top of the first electrode, and feed them via a culture medium.
Transport in the synaptic cleft
Van de Burgt worked in Eindhoven together with PhD student Setareh Kazemzadeh mainly on the transport of liquids between the two synapses. "The living cells in our system communicate with the electrode because the neurotransmitter from the cell undergoes a (redox) reaction and thus forms ions. These ions then move through the cleft to the second electrode. This changes the conductive state of this second electrode. Part of that change is retained, simulating the learning process that takes place in a real brain," Van de Burgt explains. So the processing and storage of the signal takes place at the same time, just like in a real synapse. That's what makes the system so energy-efficient.
Then the neurotransmitters must be re-formed to prepare for the next signal from the cell. This too has been imitated by the researchers. "With a microfluidic system, we were able to mimic the biologically relevant process of so-called endocytosis. This means that we can restore the synapses to their original state. We were able to achieve this by transporting oxygen to the artificial synapses," says Van de Burgt. He continues: "And that turned out to work well. It's not exactly the same process as in the brain, but it does give the same end result."
To test their synapses, the researchers used cells from rats that resemble nerve cells and that release the neurotransmitter dopamine. The researchers were able to identify this dopamine in the system. Van de Burgt: "We saw that the dopamine does indeed - as we had hoped - cause a permanent change in the second electrode, thus altering the conductive state of the system.”
Making nerves talk to each other
The next step for Van de Burgt is to really apply his system to problems in medicine. For example, to develop better prostheses that can really communicate with the body and the brain or to repair non-functioning parts of the brain with adaptive material.
But Van de Burgt also dreams about applications that are even more difficult. For example, patients with a severed spinal cord as a result of an accident. You would rather not reconnect them 1-on-1, because then the ability to learn disappears. If, instead, Van de Burgt can put an adaptive system in between, the nerves will be able to communicate with each other again. Van de Burgt: "But that's still a distant future dream, because at that point in the spinal cord you have no synapses nearby. And that's what our current system is built on."
Discussion